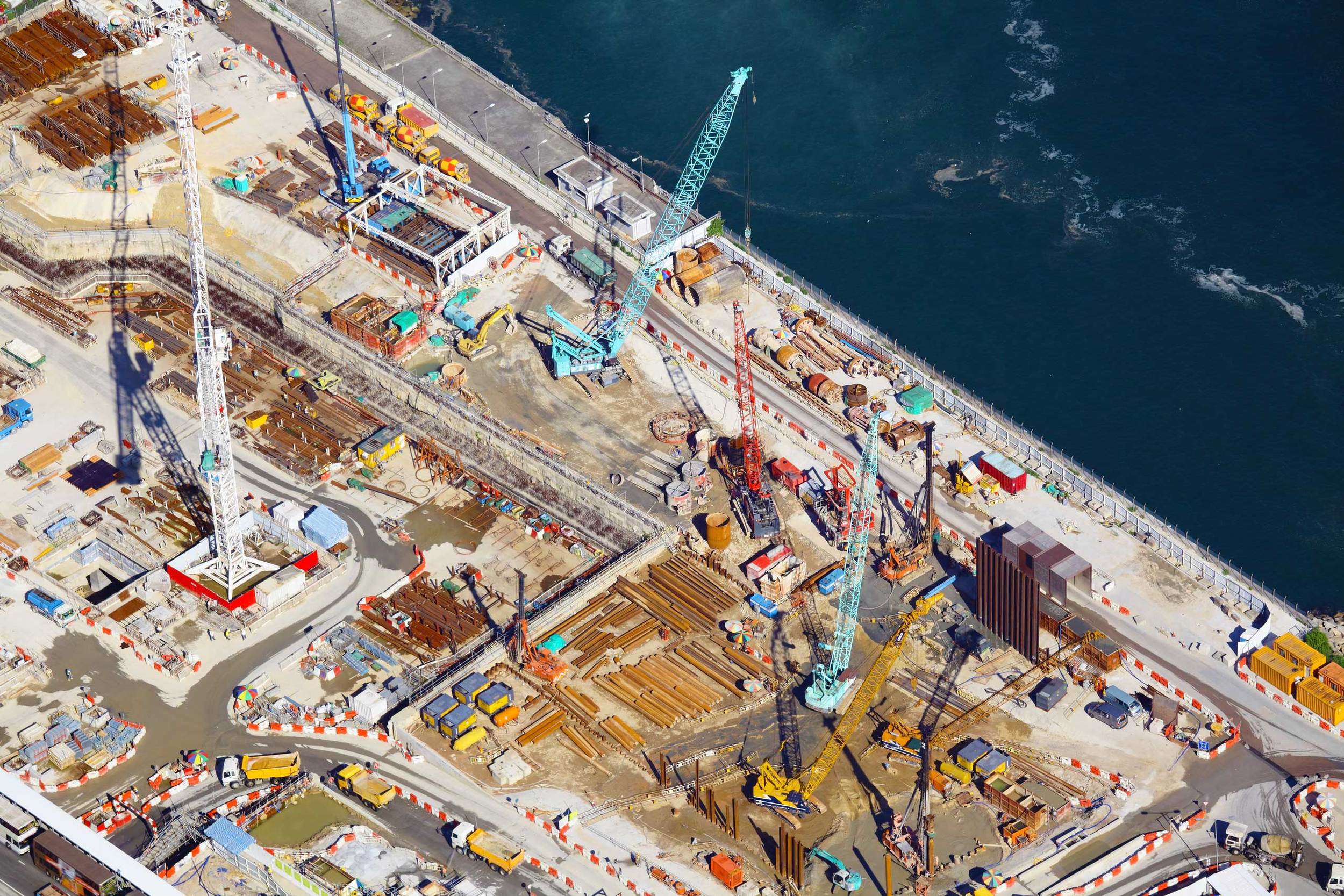
NOTES FROM THE FIELD
Rules for Average Prestress
Prestressed concrete is commonly used in many building and civil structure projects for its improved concrete performance.
Prestressed concrete is commonly used in many building and civil structure projects for its improved concrete performance. Average prestress is defined as P/A, where P is the final effective prestress force after losses and A is the cross-sectional area of the member (or design strip for two-way slabs). It is important to maintain P/A within certain defined limits. Some limits are code imposed and some are based on design experience. The minimum P/A for two-way slabs is 125 psi (0/875 MPA) as mandated by ACI 318-14. Maximum P/A is not code mandated but seen as a function of what is achievable from a practical standpoint.
In my opinion, the biggest challenge with too much P/A is increased shortening of the slab, which can lead to other challenges such as cracking due to restraint to shortening (RTS). Too much prestress can also lead to congestion and challenges fitting anchorages and anchorage zone reinforcement in the member in addition to increasing long-term prestress losses. I have developed the set of guidelines for P/A listed in Table 1.
Table 1: Pragmatic Guidelines for Average Prestress As a Function of Structure Type
Structure type | Minimum P/A, psi | Maximum P/A, psi |
---|---|---|
Parking garage slab (Zone II and III) |
||
Parking garage slab (Zone I) |
||
Parking garage beam (Zone II and III)† |
||
Parking garage beam (Zone I)† |
||
Building slab | ||
Building beam† | ||
Building transfer girder† |
Note: 1 psi = 0.007 MPa
†When calculating P/A for beams and girders, the cross-sectional area is based on the effective flange width chosen by the designer.
The maximum P/A values in Table 1 should be considered general guidelines for typical building structures that will provide reasonable results. They are not meant as absolute maximum values, as there will be occasions when it is necessary and desirable to go above these values. If you have any questions as you work with average prestressed materials, consult an expert!
Reference for definitions of zones I, II, and III:
ACI Committee 362, “Guide for the Design and Construction of Durable Concrete Parking Structures (ACI 362.1R-12),” American Concrete Institute, Farmington Hills, MI, 2012, pp. 13-14.
Load Balancing as a Design Tool
This powerful method provides a simple process for checking prestressed members using hand calculations.
Load balancing was first introduced by T.Y. Lin as an alternative method for analyzing prestressed members. This powerful method provides a simple process for checking presstressed members using hand calculations. This method of analysis seeks to remove the presstressing stands from the structural member and replace them with a set of equivalent forces that act on the member.
In traditional design, the equivalent forces act in a direction that is opposite the applied loading on the member – it is possible to “balance” a portion of the applied loads with equivalent prestress forces, sometimes referred to as the “balanced load” as referenced in Figure 1.
Figure 1 (A)
Figure 1 (B)
Figure 1 (C)
Fig. 1: Balanced load example:
(A) PT beam
(B) Free body diagram of PT tendon
(C) Net load on PT beam (Note: 1 in. = 25 mm; 1 ft = 0.3 m; 1 kip or K = 4.4 kN)
Experienced engineers recommend checking the balanced dead load percentage during design of a member as this can provide insight into the efficiency and reliability of the design. Balanced loads exceeding 100% dead load are often acceptable and even desirable, as long as the design is serviceable and code-compliant. There is no specific code requirement on the percentage of load that should be balanced with post-tensioning, and we do not recommend using a prescribed percentage as a design criterion.
We have often seen instances where designers have attempted to balance more than 300% of the dead load in some spans – this is a sure bet for failure during stressing. For transfer members such as transfer girders, transfer plates, and podium slabs, it is not unusual to have balanced loads that exceed 150% of the dead load. These cases can be complex and it is vital that the engineering team pay close attention to initial stresses, service load stresses, initial and long-term deflections, and detailing of reinforcing steel. In many transfer members; it is necessary to stage stress by stressing the member at successive intervals as load is being added to the member.
Just as overbalancing may be an indicator that the slab or beam does not have adequate thickness or depth, under-balancing may be an indicator that the member depth is overly conservative. Again, checking the percentage of dead load that is balanced is an important tool that should be used to refine and verify the design.
For typical slabs in buildings, we recommend using the values in Table 1.
Table 1: Pragmatic guidelines for balanced load range as a function of building type
Building type | Balanced load, % |
---|---|
Apartments, condominiums, and hotels (LL = 40 psf) | 40 to 70 |
Office buildings (LL > 75 psf) | 50 to 80 |
Note: 1 psf = 0.00005 MPa
In our experience, the load balancing method of analysis is perhaps the most powerful tool at the disposal of an engineer who designs prestressed structures. If you have any questions as you consider utilizing this method yourself, consult an expert!
Sidebar: Practical Hand Calculations for Post-Tensioning
by Donald Kline
While reliance on computer software for design of prestressed concrete members is nearly universal, it is possible to design these members using hand calculations. After all, this is how buildings were designed before the proliferation of computers. It is important for the design engineer to be able to perform hand calculations to check a design or even to make a last minute tendon adjustment in the field before a pour. So how is this possible? The answer lies in the load balancing method together with Equation 1.
Equation 1
Where:
- Wpre is the balanced load due to prestress (kips/ft)
- P is prestress force (kips)
- l is span length (ft)
- a is tendon drape defined in the figure (in)
Equation 1 defines the relationship between prestress force (P), tendon drape (a), and balanced load (wpre), and is based on the assumption of an idealized parabolic tendon profile. If any 2 of these variables is known, then the third can be calculated using this equation. For example, it is possible to determine the tendon force for a design strip in a two-way slab with tributary width of 20 ft, span length of 30 ft, slab thickness of 8 inches, and the assumption that the post-tensioning should balance 80 percent of the self-weight of the slab.
wpre= 0.8 x 8 in x 0.15 k/ft3 x 20 ft/12 in/ft = 1.6 k/ft
l= 30 ft
a= 8 in – 1 in – 1 in = 6 in
P= 12 x wpre x l2/8a = 360 kips
Equation 1 provides a simple and elegant way to perform preliminary design or to check a design to ensure that it is reasonable. Clearly, once the tendon force and profile are established, the design must be checked against all of the serviceability and strength requirements in the Code. But it can be shown that for members that are sized using customary span-to-depth ratios and that are subjected to typical superimposed dead and live loads, equation 1 will provide a reasonable solution. Be advised, however, that this equation does not apply for cantilevers or for spans with harped tendons. Also, it is not advisable to use Equation 1 for designing transfer members, including podium slabs. As can be seen in the example above, Equation 1 is one of the most useful tools at the disposal of engineer designing post-tensioned concrete.
Top 10 Biggest Mistakes Made in Post-Tensioned Concrete Design
While we’ve encountered many more, here’s a selection of the 10 most common mistakes we’ve encountered in PT construction.
After decades of building and inspecting in the Post-Tensioning (PT) concrete construction industry, we’ve seen the best and the worst of PT construction. And we keep a (long and growing) list of the mistakes we’ve encountered along the way. Knowing the ways and the extent to which problems can occur helps us implement the most cost-effective and long-lasting repairs possible. And most importantly, it helps us anticipate and avoid mistakes in our own work to create stronger, longer-lasting structures.
While we’ve encountered many more, here’s a selection of the 10 most common mistakes we’ve encountered in PT construction:
10. Not considering long-term cracked deflections.
PT beams and slabs have an inherent advantage over non-PT members when it comes to deflection. But it is still critical to check and correct for long-term deflection at the design stage. It is especially critical where drainage can be an issue in cases such as roofs, parking garages, and balconies, and with flat plates where long term deflections may affect window installation. 3D software will account for instantaneous deflections but long-term deflections may not be readily evident. If this is the case, take the time to create a load combination for this output.
9. Using rebar for shrinkage and temperature reinforcement in parking garage slabs.
Rebar reinforcement in this case is technically allowed by code but in our experience, a properly designed post-tensioned concrete slab will minimize cracking due to shrinkage. We recommend using PT for temperature and shrinkage reinforcement.
8. Neglecting to design general anchorage zones or delegating this responsibility.
PT suppliers are responsible for design of local anchorage zones, and many engineers incorrectly assume they will also take care of the general zone. And while code allows a licensed design professional (LDP) to delegate the design of the general anchorage zone to the contractor, we don’t recommend taking that route. The design of general anchorage zones is critical to the integrity of any post-tensioned concrete structure and we recommend that the LDP complete this task personally.
7. Sweeping tendons at low points without proper detailing.
If it is necessary to sweep tendons laterally – and often, it will be as a result of miss-aligned columns – make sure you are detailing these areas properly, whether at low points or at any other point in the slab. Tendons with lateral curvature at low points have a tendency to blowout the bottom of the slab – either immediately upon stressing or many years later. Refer to the PTI Manual, Sixth Edition, 2006, p. 125 for more information on proper detailing for this condition.
6. Ignoring the effect of slab folds on tendon profile.
At slab folds, it is important to check tendon profile at critical points along the span to ensure the cable is positioned properly. It is not uncommon to see a theoretical tendon path falling outside of the slab at the fold – clearly a situation to be avoided. But it is just as important to examine locations where reverse curvature with minimum cover could lead to pop-outs during stressing.
5. Overbalancing dead load in your designs.
There is no specific code requirement for how much load to balance with PT – but checking the balanced load is good practice. Balanced loads exceeding 100% DL are often acceptable and even desirable, so long as the design is serviceable and code-compliant. But we have seen designers balance more than 400% DL in some spans, which almost always leads to sudden failure of the slab.
4. Reliance on 3D finite element software for design and drawings.
Finite element design packages can be incredibly useful in the design of PT structures. We use them frequently ourselves. But in the hands of an inexperienced designer, such programs can lead to lazy design habits, poor quality drawings, and bad designs. Always have an experienced PT designer review the computer’s results and never use drawings produced by a computer program without thorough review.
3. Poor specifications related to finishing and inspection of tendon ends.
The finishing process includes cutting strand tails, installing the protective end cap, cleaning the stressing pocket, and patching the pocket with high quality mortar. This is one of the most important steps in ensuring the durability of the structure. When tendon ends are improperly finished, structural durability is compromised. It is critical that the specification include inspection of tendon finishing in the inspector’s responsibilities.
2. Failing to specify encapsulated systems.
Encapsulated PT systems should be specified on all PT buildings (with possible exception of slab on ground construction). This is now mandated by ACI, and we believe it to be good practice.
1. Ignoring restraint to shortening effects.
This is the source of most problems with new designs – both aesthetic and structural. Stiff vertical elements in the structure such as columns and walls have a tendency to restrain shortening of the PT member. Shortening is caused by elastic shortening, drying shrinkage, and creep. It is critical to evaluate the design in terms of potential for restraint and include measures to mitigate such effects.
In our experience, these mistakes are often the result of an even bigger mistake – a failure to plan ahead! Give yourself and your team enough time to get the job done right. And when in doubt – consult a seasoned expert!
8 Signs That Your Garage is in Need of Repair
Here are some tell-tale signs that your garage is in need of repair.
At Kline Engineering, we have decades of experience identifying and repairing structural distress. But for property managers or owners, distinguishing between normal wear and tear in your parking structure and serious structural distress can be tricky. This is because symptoms of serious deterioration can often appear to be little more than cosmetic damage to the untrained eye. And misidentifying structural damage can quickly degrade the capacity and integrity of your garage!
Don’t panic! Learn to recognize some of the most common signs of structural failure and when in doubt, contact a professional.
Here are some tell-tale signs that your garage is in need of repair:
1. Spalling of the top surface of the concrete slab.
When embedded reinforcing steel corrodes, it expands to more than twice its original volume. This expansion creates tensile forces within the concrete surface leading to spalling and delamination of the concrete. This is an indication that the concrete passive layer (protective layer) has been degraded leading to corrosion of the embedded steel. Left unchecked, the durability of the concrete is severely degraded.
2. Exposed steel in the top surface of the concrete slab.
Concrete protects the embedded steel reinforcement from corrosion. Exposed steel indicates reduced concrete cover, which may be widespread, thus reducing the thickness of the protective concrete cover, leading to reduced durability.
3. Cracks large enough that water leaks through.
Cracks in concrete are caused by a variety of factors. You may have heard the statement “all concrete cracks”. Generally, this statement is correct; however, one must discern the difference between an acceptable crack and one that may lead to durability issues. Cracks that are large enough for water to leak through the deck should be repaired.
4. White mineral efflorescence hanging from cracks in the slab soffit (ceiling).
Efflorescence is the result of soluble salts migrating to the surface of the concrete where the water evaporates, depositing a whitish trail of crystalline salts. Efflorescence itself is harmless, but it is usually a sign that water is finding its way into the concrete. This can lead to corrosion of the embedded steel.
5. White efflorescence stains on the vertical faces of beams and slabs.
This can be a symptom of water infiltration into the post-tensioning system leading to corrosion of the PT. Left untreated, it can lead to failure of the PT system.
6. Grease stains not on the top of the slab, but on the bottom (soffit) of the slab.
The tendons within a post-tension concrete structure are protected from corrosion with grease and plastic sheathing. Visible grease stains on the bottom of a slab might indicate that the protection of the post-tensioning system has been breached.
7. Loose vehicular barrier cables.
Loose barrier cables indicate that the cable end anchors are failing. This is a serious safety risk as vehicles that impact the cables will not be restrained from falling. Several fatalities have occurred when vehicles have fallen from parking structures.
8. Ponding water on the top surface of the slab after rain or snow.
A properly constructed parking slab is designed to drain easily, but over time, the natural sagging or “creep” of a concrete structure can lead to ponding in new areas. This will lead to water damage and reduced durability.
These are not the only signs of structural damage but they are by far the most common. Learning to recognize them can be a great early warning system. The earlier you can identify the warning signs of structural damage, the more quickly and accurately repairs can be done. But remember – when in doubt, consult an expert!